Chapter 3 - The Quantum Horizon: When Classical Meets Quantum
- pranavajoshi8
- Feb 25
- 12 min read
Updated: Mar 6
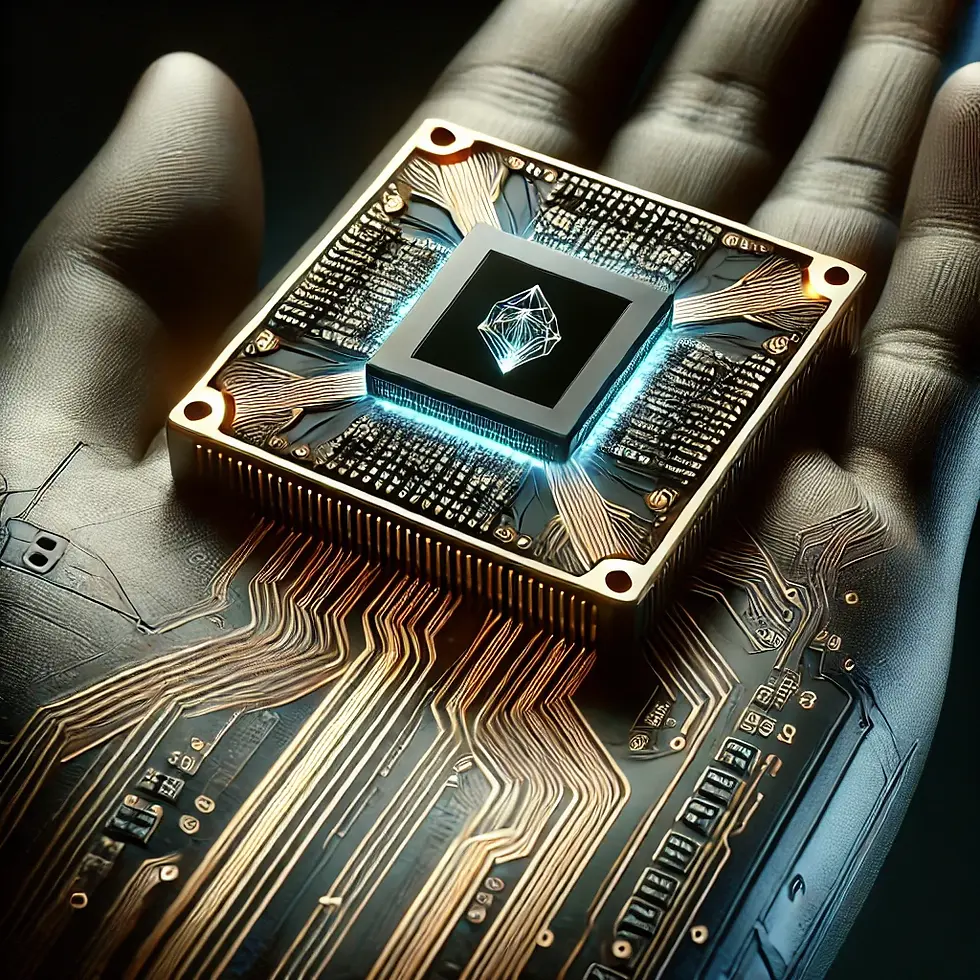
In our previous chapters, we explored the accelerating pace of technological change and examined how AI differs fundamentally from previous revolutions. Now, we turn our attention to another paradigm-shifting technology that may transform our world just as profoundly: quantum computing.
While AI has captured public imagination through its visible impacts on everyday life, quantum computing has largely remained in the realm of researchers and specialized industries. Yet, the convergence of these two technologies could trigger the next great technological leap, reshaping everything from medicine to climate science to cybersecurity [1].
Beyond Binary: The Quantum Computing Revolution
To understand what makes quantum computing revolutionary, we must first understand its fundamental differences from classical computing.
All digital technology since the invention of the computer has operated on the same basic principle: binary information processing. Whether it's your smartphone, laptop, or the most powerful supercomputer, classical computing relies on bits that can be either 0 or 1, nothing in between. This binary foundation served us well, powering the digital revolution that transformed our world [2].
Quantum computing, however, breaks this paradigm entirely. Instead of bits, quantum computers use quantum bits, or qubits. Thanks to the strange properties of quantum mechanics, qubits can exist in multiple states simultaneously—a phenomenon called superposition. Even more remarkably, qubits can be entangled with each other, creating interconnections that allow quantum computers to process information in ways that defy our everyday understanding of reality [3].
The result? For certain problems, quantum computers could solve in seconds what might take classical computers thousands of years. This isn't merely an incremental improvement—it's a fundamental reimagining of computation itself.
A Brief History: From Thought Experiments to Quantum Machines
Quantum computing didn't emerge overnight. Its journey from theoretical concept to physical reality spans decades of scientific breakthroughs and engineering innovations.
The birth of quantum computing as a concept is often attributed to Richard Feynman, who in 1981 proposed that quantum mechanics could be used to perform computations [4]. This insight sparked a new field of research, but significant hurdles remained. Quantum systems are notoriously fragile—susceptible to noise, temperature fluctuations, and environmental interference. Even the slightest disturbance can cause "decoherence," destroying the quantum states necessary for computation [5].
For decades, quantum computers remained largely theoretical. But persistent innovation has yielded remarkable progress. In 2019, Google claimed to achieve "quantum supremacy" when its 53-qubit Sycamore processor performed a calculation in minutes that would take the world's most powerful supercomputer thousands of years. While debates continue about this milestone, it clearly signaled a new era in quantum computing.
Today, companies like IBM, Google, Microsoft, and startups like Rigetti and IonQ are rapidly advancing quantum hardware and software. IBM's most advanced quantum processor now exceeds 1,000 qubits, though challenges with error rates and coherence times remain significant obstacles to practical applications [7].
In a major development, Microsoft CEO Satya Nadella recently unveiled the company's topological quantum computer, Majorana-1. This demonstration represents a potentially groundbreaking approach to quantum computing based on topological qubits, which are theoretically more stable and resistant to errors than other qubit technologies. According to Nadella, Microsoft has taken a "different, longer path" to quantum computing, focusing on a more reliable foundation that could ultimately enable more practical quantum applications by addressing the critical challenge of quantum error correction. While still in early stages, this development marks a significant milestone in Microsoft's quantum roadmap.
Where Quantum Computing Could Transform Our World
The transformative potential of quantum computing spans numerous domains, but a few stand out as particularly promising:
1. Drug Discovery and Materials Science
Perhaps the most immediate impact of quantum computing will be in simulating molecular interactions. Classical computers struggle to model the quantum behavior of molecules, making drug discovery a slow, expensive process of trial and error.
Quantum computers, by contrast, are perfectly suited for this task. They can simulate molecular behavior at the quantum level, potentially revolutionizing how we develop new medicines, catalysts, and materials [9].
"Quantum computers can represent molecular interactions directly, potentially reducing the time to discover new drugs from years to days." - Dr. Dario Gil, IBM Research [10]
Companies like Zapata Computing are already partnering with pharmaceutical firms to apply quantum algorithms to drug discovery. As quantum hardware improves, we could see dramatic acceleration in developing treatments for cancer, Alzheimer's, and other diseases that have resisted traditional approaches.
2. Climate Modeling and Energy
Climate models are among the most complex simulations run on classical supercomputers. Even so, they rely on approximations that limit their accuracy and granularity.
Quantum computing could enable far more precise climate models by efficiently processing the vast number of variables involved [12]. This could lead to more accurate climate predictions and better strategies for mitigation and adaptation.
Similarly, quantum computing could help develop more efficient solar cells, batteries, and superconductors by simulating their properties at the quantum level. The potential impact on renewable energy and sustainability could be enormous [13].
3. Cryptography and Security
Perhaps the most double-edged application of quantum computing lies in cryptography. Most modern encryption relies on the difficulty of factoring large numbers—a task that classical computers find extraordinarily time-consuming but that quantum computers (using Shor's algorithm) could potentially perform quickly [14].
This poses a serious threat to current security systems, from banking to national defense. As a result, governments and companies worldwide are racing to develop "post-quantum cryptography"—encryption methods that can withstand quantum attacks [15].
At the same time, quantum technology offers new forms of secure communication through quantum key distribution (QKD), which uses the principles of quantum mechanics to detect any attempt at eavesdropping on a communication channel [16].
The Convergence: When Quantum Meets AI
While quantum computing and artificial intelligence are revolutionary on their own, their convergence could be even more transformative. This nascent field of quantum machine learning sits at the intersection of quantum physics, computer science, and artificial intelligence [17].
Quantum computers could potentially accelerate certain machine learning tasks, including:
Training complex neural networks: Quantum algorithms might dramatically speed up the training of AI models by optimizing high-dimensional parameter spaces more efficiently [18].
Solving reinforcement learning problems: Quantum computing could help AI systems find optimal solutions more quickly in complex environments with large state spaces [19].
Processing quantum data: As quantum sensors become more common, quantum computers would be the natural choice for processing the quantum data they generate [20].
Meanwhile, AI is already helping advance quantum computing by optimizing quantum circuit design, error correction, and control systems. This mutually beneficial relationship could accelerate progress in both fields [21].
Microsoft's Azure Quantum, Google's Quantum AI, and IBM's Quantum Experience are already exploring these synergies, developing tools that integrate classical AI with quantum processing capabilities [22].
The Skills Gap: Preparing for a Quantum Future
The quantum revolution brings enormous opportunities, but also presents a significant skills challenge. Quantum computing requires a unique interdisciplinary skill set spanning physics, computer science, mathematics, and specific domain knowledge [24].
This skills gap represents both a challenge and an opportunity. Universities worldwide are establishing quantum information science programs, while companies like IBM and Google offer online courses and certification programs.
For those looking to enter the field, several pathways exist:
Academic route: Advanced degrees in physics, computer science, or mathematics with a specialization in quantum information [25]
Industry training: Certification programs from technology companies combined with hands-on experience with quantum programming frameworks [26]
Self-directed learning: Online resources like Qiskit Textbook, Microsoft's Quantum Katas, and platforms like edX and Coursera offer accessible entry points [27]
What's notable about the quantum computing field is its relative youth and openness. Unlike more established technologies, the quantum space still has many fundamental problems to solve, creating opportunities for newcomers to make significant contributions.
The Timeframe: When Will Quantum Computing Impact Society?
Despite rapid progress, significant hurdles remain before quantum computing becomes a mainstream technology:
Error correction: Quantum bits are highly susceptible to errors, requiring complex error-correction techniques that consume significant qubit resources [28]
Scaling challenges: Building large-scale, fault-tolerant quantum computers requires solving formidable engineering challenges [29]
Algorithmic development: We're still discovering what problems are best suited for quantum advantage [30]
Most experts predict a gradual progression rather than a sudden revolution:
Near-term (3-5 years): Early commercial applications in specific niches, primarily used alongside classical systems [31]
Mid-term (5-10 years): Fault-tolerant quantum computers with hundreds of logical qubits, enabling more practical applications in chemistry and optimization [32]
Long-term (10+ years): Widespread quantum advantage across multiple industries, with thousands of logical qubits enabling transformative applications [33]
This timeline aligns with recent statements from industry leaders. Google CEO Sundar Pichai, speaking in February 2025, estimated that practical quantum computing applications are "five to ten years away." Pichai emphasized that while Google has made significant progress in quantum hardware and demonstrating quantum advantage for specialized problems, the technology still requires substantial development before it can deliver consistent, practical value across industries [34]. He highlighted continued investment in error correction as a critical focus area, noting that "quantum utility" -- where quantum computers reliably outperform classical systems on commercially relevant problems -- remains the key milestone the industry is working toward.
As with previous technological revolutions, the most transformative applications may be ones we haven't yet envisioned. When classical computers were first developed, few predicted smartphones, social media, or cloud computing. Similarly, quantum computing may enable entirely new paradigms we can scarcely imagine today.
Democratizing Quantum Knowledge: Beyond the Experts
As quantum computing evolves from research labs to commercial applications, a key challenge will be democratizing knowledge and access. Unlike earlier technological revolutions that could be understood through intuitive metaphors (like the industrial revolution's mechanical systems), quantum computing operates on principles that seem to contradict our everyday experience of reality [41].
This creates a potential knowledge divide that could exacerbate existing social inequalities. The most successful organizations and individuals will be those who can translate quantum concepts into practical applications without requiring everyone to understand the underlying quantum mechanics [42].
Educational institutions, from K-12 schools to universities and corporate training programs, will need to develop new approaches for teaching quantum concepts that don't require advanced mathematics or physics backgrounds [44]. The goal isn't to turn everyone into quantum physicists, but to create quantum-literate professionals who can identify opportunities for quantum applications in their domains.
The Global Race for Quantum Advantage
Quantum computing has also become a focal point of global technological competition. The United States, China, European Union, Japan, and other nations have launched multi-billion-dollar national quantum initiatives, recognizing the strategic importance of leadership in this field [45].
This geopolitical dimension adds urgency to quantum development but also raises concerns about access, standards, and the militarization of quantum technology. International cooperation will be essential to establish norms and frameworks that maximize the benefits of quantum computing while minimizing risks [46].
Societal Implications: Navigating the Quantum Future
The quantum revolution raises important societal questions:
Security and privacy: How do we maintain secure communications in a post-quantum world? [36]
Economic impact: Which industries will be disrupted first, and how can workers and businesses adapt? [37]
Access and equity: How do we ensure quantum technology benefits humanity broadly rather than concentrating power? [38]
Ethical considerations: What ethical frameworks should guide the development of technologies that could, for example, break encryption protecting sensitive personal data? [39]
These questions require proactive consideration from policymakers, industry leaders, and civil society. Several initiatives are already addressing these concerns, including the World Economic Forum's Quantum Computing Governance initiative and the Quantum Industry Coalition [40].
Personal Adaptation Strategies
For individuals navigating this changing technological landscape, there are several key strategies to remain relevant and capitalize on emerging opportunities:
Develop quantum literacy: Understand the basic principles of quantum computing and how they might apply to your field, even if you don't need to master the mathematical details [48].
Identify transition points: Watch for signs that quantum computing is approaching practical applications in your industry, such as early experimental results or increasing investment [49].
Focus on complementary skills: Develop expertise in areas that will complement quantum computing, such as domain knowledge that helps translate quantum capabilities into practical applications [50].
Join quantum communities: Participate in online forums, conferences, and user groups to stay current on developments and build a professional network in the quantum ecosystem [51].
Experiment with current tools: Platforms like IBM Quantum Experience, Amazon Braket, and Microsoft's Azure Quantum allow anyone to begin experimenting with quantum programming today, even with limited technical background [52].
Conclusion: Preparing for the Next Revolution
Quantum computing represents a fundamental shift in how we process information—as significant as the leap from mechanical calculators to digital computers. While the technology is still maturing, its potential impact on society, work, and human progress is too great to ignore [53].
As with AI, the question is not whether quantum computing will transform our world, but how we will navigate that transformation. By understanding quantum computing's potential, developing appropriate skills, and thoughtfully addressing the societal implications, we can help ensure that this technology serves humanity's best interests [54].
The convergence of quantum computing with AI and other emerging technologies will likely create entirely new fields and opportunities that we can scarcely imagine today. The most successful professionals will be those who remain curious, adaptable, and willing to continuously learn as these technologies evolve.
In our next chapter, we'll explore the tangible manifestation of these digital revolutions—examining how AI and emerging technologies are reshaping the physical products we interact with daily, creating new paradigms in human-machine interfaces.
In our next chapter, we'll explore the tangible manifestation of these digital revolutions—examining how AI and emerging technologies are reshaping the physical products we interact with daily, creating new paradigms in human-machine interfaces.
References
National Science Foundation. (2023). "Convergent Applications of Quantum Computing and Artificial Intelligence." NSF Strategic Research Initiatives Report.
National Institute of Standards and Technology. (2022). "Evolution of Computing: From Classical to Quantum." NIST Special Publication 800-207.
MIT Technology Review. (2023). "Quantum Computing: Understanding the Core Principles." MIT Press.
Feynman, R. P. (1982). "Simulating physics with computers." International Journal of Theoretical Physics, 21(6), 467-488.
Harvard Quantum Initiative. (2023). "Quantum Decoherence: Challenges and Solutions." Harvard University Press.
Arute, F., Arya, K., Babbush, R. et al. (2019). "Quantum supremacy using a programmable superconducting processor." Nature, 574, 505-510.
IBM Research. (2024). "IBM Quantum Development Roadmap." IBM Technical White Paper.
Microsoft Research. (2025). "Topological Quantum Computing: The Path to Fault Tolerance." Microsoft Technical Report.
Stanford Institute for Theoretical Physics. (2023). "Quantum Simulation for Drug Discovery." Stanford University Research Brief.
Gil, D. (2022). "The business of quantum computing." Harvard Business Review, 100(1), 104-111.
University of Toronto & Menten AI. (2023). "Accelerating Drug Discovery through Quantum Computing." Journal of Computational Chemistry.
Massachusetts Institute of Technology. (2024). "Quantum Computing Applications in Climate Modeling." MIT Energy Initiative Report.
Princeton University. (2023). "Quantum Algorithms for Energy Materials Design." Princeton Materials Institute.
National Security Agency. (2023). "Preparing for Post-Quantum Cryptography." NSA Cybersecurity Advisory.
National Institute of Standards and Technology. (2024). "Post-Quantum Cryptography Standardization." NIST Interagency Report 8413.
CERN Quantum Technology Initiative. (2023). "Quantum Key Distribution: Theory and Implementation." CERN Yellow Reports.
MIT-IBM Watson AI Lab. (2024). "The Convergence of Quantum Computing and Artificial Intelligence." Research Monograph.
Google AI Quantum. (2023). "Quantum Neural Networks: Theory and Applications." Google Research.
Stanford University Institute for Human-Centered Artificial Intelligence. (2024). "Reinforcement Learning with Quantum Advantage." HAI Working Paper.
University of California, Berkeley. (2023). "Quantum Sensors and Processing: A New Paradigm." Berkeley Quantum Information Science Technical Report.
Carnegie Mellon University. (2024). "AI-Assisted Quantum Computing: Accelerating Progress." CMU Quantum Computing Center.
Deloitte Center for Quantum Technology. (2024). "Enterprise Quantum Computing: Platforms and Applications." Deloitte Insights.
McKinsey Global Institute. (2024). "The Quantum Technology Job Market: 2025-2030." McKinsey Quarterly Report.
National Science Foundation. (2023). "Quantum Information Science Education Challenges and Opportunities." NSF Education Directorate.
University of Chicago. (2024). "Academic Pathways to Quantum Careers." Pritzker School of Molecular Engineering Report.
IBM Quantum Workforce Development. (2023). "Industry Training for the Quantum Era." IBM Skills Academy.
MIT OpenCourseWare. (2023). "Self-Directed Learning in Quantum Computing: Resources and Strategies." MIT Open Learning.
California Institute of Technology. (2023). "Quantum Error Correction: State of the Art." Caltech Institute for Quantum Information and Matter.
Yale Quantum Institute. (2024). "Engineering Challenges in Scalable Quantum Computers." Yale University Press.
Oxford Quantum Computing Center. (2023). "Quantum Algorithmic Development: Progress and Challenges." Oxford University Research Report.
Boston Consulting Group. (2023). "The Commercial Timeline for Quantum Computing." BCG Henderson Institute Report.
Harvard Business School. (2024). "Quantum Computing Investment Horizons: 2025-2035." HBS Digital Initiative.
World Economic Forum. (2024). "Future of Quantum Computing: Scenarios Through 2040." WEF Future of Technology Series.
The Quantum Insider. (2025). "Practical Quantum Computing Five to Ten Years Away: Google CEO." Retrieved from Quantum Insider Research Report.
Boston Consulting Group. (2023). "Where Will Quantum Computers Create Value—and When?" BCG Technology Advantage Report.
Wharton School of Business. (2024). "Quantum Computing and the Future of Digital Security." Knowledge@Wharton Special Report.
Stanford Digital Economy Lab. (2024). "Quantum Computing's Economic Disruption Potential." Stanford Institute for Economic Policy Research.
Brookings Institution. (2023). "Ensuring Equitable Access to Quantum Technologies." Brookings Technology and Innovation Report.
Princeton Center for Human Values. (2023). "Ethical Frameworks for Quantum Technology Development." Princeton University Ethics Series.
World Economic Forum. (2022). "Quantum Computing Governance Principles." Global Technology Governance Report.
Massachusetts Institute of Technology. (2023). "Making Quantum Concepts Accessible." MIT OpenCourseWare Initiative Report.
Brookings Institution. (2024). "Bridging the Quantum Divide: Ensuring Equitable Access to Next-Generation Computing." Brookings Technology and Innovation Report.
Quantum Economic Development Consortium. (2024). "Workforce Development in the Quantum Era." QED-C Industry Research Report.
National Science Foundation. (2023). "Quantum Education for the 21st Century." NSF STEM Education Advisory Report.
Center for Strategic and International Studies. (2024). "National Quantum Initiatives: A Global Analysis." CSIS Technology Policy Program.
Carnegie Endowment for International Peace. (2023). "International Cooperation in Quantum Technology Development." Global Technology and Security Program.
McKinsey Global Institute. (2024). "The Geopolitics of Quantum Technologies." McKinsey Quarterly Special Edition.
Harvard Extension School. (2024). "Quantum Literacy for Professionals." Professional Development Series.
Wharton School of Business. (2023). "Identifying Industry Transition Points in Quantum Computing." Knowledge@Wharton Technology Series.
Stanford University. (2024). "Complementary Skills for the Quantum Economy." Stanford Digital Economy Lab.
MIT Sloan School of Management. (2023). "Building Professional Networks in Emerging Technologies." MIT Sloan Management Review.
U.S. Department of Energy Office of Science. (2024). "Democratizing Access to Quantum Computing Resources." National Quantum Information Science Research Centers.
World Economic Forum. (2023). "The Next Computing Revolution: Preparing for the Quantum Future." WEF Future of Technology Series.
National Academies of Sciences, Engineering, and Medicine. (2024). "Societal Implications of Quantum Information Science and Technology." Consensus Study Report.
Appendix: Carrer Transition Guide Toolkit
Comentarios